About IPv6
IPv6, which is designed to replace IPv4, increases the number of network address bits from 32 bits (in IPv4) to 128 bits. IPv6 is based on IPv4, but it includes a much larger address space and other improvements such as a simplified main header and extension headers.
The larger IPv6 address space allows networks to scale and provide global reachability. The simplified IPv6 packet header format handles packets more efficiently. The flexibility of the IPv6 address space reduces the need for private addresses and the use of Network Address Translation (NAT), which translates private (not globally unique) addresses into a limited number of public addresses. IPv6 enables new application protocols that do not require special processing by border routers at the edge of networks.
IPv6 functionality, such as prefix aggregation, simplified network renumbering, and IPv6 site multihoming capabilities, enables more efficient routing. IPv6 supports Routing Information Protocol (RIP), Integrated Intermediate System-to-Intermediate System (IS-IS), Open Shortest Path First (OSPF) for IPv6, and multiprotocol Border Gateway Protocol (BGP).
IPv6 Address Formats
An IPv6 address has 128 bits or 16 bytes. The address is divided into eight, 16-bit hexadecimal blocks separated by colons (:) in the format x:x:x:x:x:x:x:x.
Two examples of IPv6 addresses are as follows:
2001:0DB8:7654:3210:FEDC:BA98:7654:3210
2001:0DB8:0:0:8:800:200C:417A
IPv6 addresses contain consecutive zeros within the address. You can use two colons (::) at the beginning, middle, or end of an IPv6 address to replace the consecutive zeros. The following table shows a list of compressed IPv6 address formats.
![]() Note |
You can use two colons (::) only once in an IPv6 address to replace the longest string of consecutive zeros within the address. |
You can use a double colon as part of the IPv6 address when consecutive 16-bit values are denoted as zero. You can configure multiple IPv6 addresses per interface but only one link-local address.
The hexadecimal letters in IPv6 addresses are not case sensitive.
IPv6 Address Type | Preferred Format | Compressed Format |
---|---|---|
Unicast |
2001:0:0:0:0:DB8:800:200C:417A |
2001::0DB8:800:200C:417A |
Multicast |
FF01:0:0:0:0:0:0:101 |
FF01::101 |
Loopback |
0:0:0:0:0:0:0:0:1 |
::1 |
Unspecified |
0:0:0:0:0:0:0:0:0 |
:: |
A node may use the loopback address listed in the table to send an IPv6 packet to itself. The loopback address in IPv6 is the same as the loopback address in IPv4. For more information, see Overview.
![]() Note |
You cannot assign the IPv6 loopback address to a physical interface. A packet that contains the IPv6 loopback address as its source or destination address must remain within the node that created the packet. IPv6 routers do not forward packets that have the IPv6 loopback address as their source or destination address. |
![]() Note |
You cannot assign an IPv6 unspecified address to an interface. You should not use the unspecified IPv6 addresses as destination addresses in IPv6 packets or the IPv6 routing header. |
The IPv6 prefix is in the form documented in RFC 2373 where the IPv6 address is specified in hexadecimal using 16-bit values between colons. The prefix length is a decimal value that indicates how many of the high-order contiguous bits of the address comprise the prefix (the network portion of the address). For example, 2001:0DB8:8086:6502::/32 is a valid IPv6 prefix.
IPv6 Unicast Addresses
An IPv6 unicast address is an identifier for a single interface on a single node. A packet that is sent to a unicast address is delivered to the interface identified by that address.
Aggregatable Global Addresses
An aggregatable global address is an IPv6 address from the aggregatable global unicast prefix. The structure of aggregatable global unicast addresses enables strict aggregation of routing prefixes that limits the number of routing table entries in the global routing table. Aggregatable global addresses are used on links that are aggregated upward through organizations and eventually to the Internet service providers (ISPs).
Aggregatable global IPv6 addresses are defined by a global routing prefix, a subnet ID, and an interface ID. Except for addresses that start with binary 000, all global unicast addresses have a 64-bit interface ID. The IPv6 global unicast address allocation uses the range of addresses that start with binary value 001 (2000::/3). The following figure shows the structure of an aggregatable global address.

Addresses with a prefix of 2000::/3 (001) through E000::/3 (111) are required to have 64-bit interface identifiers in the extended universal identifier (EUI)-64 format. The Internet Assigned Numbers Authority (IANA) allocates the IPv6 address space in the range of 2000::/16 to regional registries.
The aggregatable global address consists of a 48-bit global routing prefix and a 16-bit subnet ID or Site-Level Aggregator (SLA). In the IPv6 aggregatable global unicast address format document (RFC 2374), the global routing prefix included two other hierarchically structured fields called Top-Level Aggregator (TLA) and Next-Level Aggregator (NLA). The IETF decided to remove the TLS and NLA fields from the RFCs because these fields are policy based. Some existing IPv6 networks deployed before the change might still use networks that are on the older architecture.
A subnet ID, which is a 16-bit subnet field, can be used by individual organizations to create a local addressing hierarchy and to identify subnets. A subnet ID is similar to a subnet in IPv4, except that an organization with an IPv6 subnet ID can support up to 65,535 individual subnets.
An interface ID identifies interfaces on a link. The interface ID is unique to the link. In many cases, an interface ID is the same as or based on the link-layer address of an interface. Interface IDs used in aggregatable global unicast and other IPv6 address types have 64 bits and are in the modified EUI-64 format.
Interface IDs are in the modified EUI-64 format in one of the following ways:
-
For all IEEE 802 interface types (for example, Ethernet and Fiber Distributed Data interfaces), the first three octets (24 bits) are the Organizationally Unique Identifier (OUI) of the 48-bit link-layer address (MAC address) of the interface, the fourth and fifth octets (16 bits) are a fixed hexadecimal value of FFFE, and the last three octets (24 bits) are the last three octets of the MAC address. The Universal/Local (U/L) bit, which is the seventh bit of the first octet, has a value of 0 or 1. Zero indicates a locally administered identifier; 1 indicates a globally unique IPv6 interface identifier.
-
For all other interface types (for example, serial, loopback, ATM, and Frame Relay types), the interface ID is similar to the interface ID for IEEE 802 interface types; however, the first MAC address from the pool of MAC addresses in the router is used as the identifier (because the interface does not have a MAC address).
Note
For interfaces that use the Point-to-Point Protocol (PPP), where the interfaces at both ends of the connection might have the same MAC address, the interface identifiers at both ends of the connection are negotiated (picked randomly and, if necessary, reconstructed) until both identifiers are unique. The first MAC address in the router is used as the identifier for interfaces using PPP.
If no IEEE 802 interface types are in the router, link-local IPv6 addresses are generated on the interfaces in the router in the following sequence:
-
The router is queried for MAC addresses (from the pool of MAC addresses in the router).
-
If no MAC addresses are available in the router, the serial number of the router is used to form the link-local addresses.
-
If the serial number of the router cannot be used to form the link-local addresses, the router uses a Message Digest 5 (MD5) hash to determine the MAC address of the router from the hostname of the router.
Link-Local Addresses
A link-local address is an IPv6 unicast address that can be automatically configured on any interface using the link-local prefix FE80::/10 (1111 1110 10) and the interface identifier in the modified EUI-64 format. Link-local addresses are used in the Neighbor Discovery Protocol (NDP) and the stateless autoconfiguration process. Nodes on a local link can use link-local addresses to communicate; the nodes do not need globally unique addresses to communicate. The figure shows the structure of a link-local address.
IPv6 routers cannot forward packets that have link-local source or destination addresses to other links.
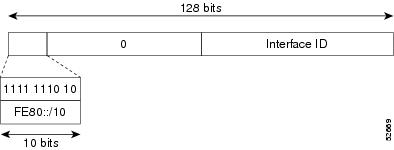
IPv4-Compatible IPv6 Addresses
An IPv4-compatible IPv6 address is an IPv6 unicast address that has zeros in the high-order 96 bits of the address and an IPv4 address in the low-order 32 bits of the address. The format of an IPv4-compatible IPv6 address is 0:0:0:0:0:0:A.B.C.D or ::A.B.C.D. The entire 128-bit IPv4-compatible IPv6 address is used as the IPv6 address of a node, and the IPv4 address embedded in the low-order 32 bits is used as the IPv4 address of the node. IPv4-compatible IPv6 addresses are assigned to nodes that support both the IPv4 and IPv6 protocol stacks and are used in automatic tunnels. The figure shows the structure of a n IPv4-compatible IPv6 address and a few acceptable formats for the address.

Unique Local Addresses
A unique local address is an IPv6 unicast address that is globally unique and is intended for local communications. It is not expected to be routable on the global Internet and is routable inside of a limited area, such as a site, and it may be routed between a limited set of sites. Applications might treat unique local addresses like global scoped addresses.
A unique local address has the following characteristics:
-
It has a globally unique prefix (it has a high probability of uniqueness).
-
It has a well-known prefix to allow for easy filtering at site boundaries.
-
It allows sites to be combined or privately interconnected without creating any address conflicts or requiring renumbering of interfaces that use these prefixes.
-
It is ISP-independent and can be used for communications inside of a site without having any permanent or intermittent Internet connectivity.
-
If it is accidentally leaked outside of a site through routing or the Domain Name Server (DNS), there is no conflict with any other addresses.
The figure shows the structure of a unique local address.

Site Local Addresses
Because RFC 3879 deprecates the use of site-local addresses, you should follow the recommendations of unique local addressing (ULA) in RFC 4193 when you configure private IPv6 addresses.
IPv4 Packet Header
The base IPv4 packet header has 12 fields with a total size of 20 octets (160 bits). The 12 fields may be followed by an Options field, which is followed by a data portion that is usually the transport-layer packet. The variable length of the Options field adds to the total size of the IPv4 packet header. The shaded fields of the IPv4 packet header are not included in the IPv6 packet header.

Simplified IPv6 Packet Header
The base IPv6 packet header has 8 fields with a total size of 40 octets (320 bits). Fragmentation is handled by the source of a packet, and checksums at the data link layer and transport layer are used. The User Datagram Protocol (UDP) checksum checks the integrity of the inner packet, and the base IPv6 packet header and Options field are aligned to 64 bits, which can facilitate the processing of IPv6 packets.
The table lists the fields in the base IPv6 packet header.
Field | Description |
---|---|
Version |
Similar to the Version field in the IPv4 packet header, except that the field lists number 6 for IPv6 instead of number 4 for IPv4. |
Traffic Class |
Similar to the Type of Service field in the IPv4 packet header. The Traffic Class field tags packets with a traffic class that is used in differentiated services. |
Flow Label |
New field in the IPv6 packet header. The Flow Label field tags packets with a specific flow that differentiates the packets at the network layer. |
Payload Length |
Similar to the Total Length field in the IPv4 packet header. The Payload Length field indicates the total length of the data portion of the packet. |
Next Header |
Similar to the Protocol field in the IPv4 packet header. The value of the Next Header field determines the type of information that follows the base IPv6 header. The type of information that follows the base IPv6 header can be a transport-layer packet (for example, a TCP or UDP packet) or an Extension Header, as shown in the figure below. |
Hop Limit |
Similar to the Time to Live field in the IPv4 packet header. The value of the Hop Limit field specifies the maximum number of routers that an IPv6 packet can pass through before the packet is considered invalid. Each router decrements the value by one. Because no checksum is in the IPv6 header, the router can decrement the value without needing to recalculate the checksum, which saves processing resources. |
Source Address |
Similar to the Source Address field in the IPv4 packet header, except that the field contains a 128-bit source address for IPv6 instead of a 32-bit source address for IPv4. |
Destination Address |
Similar to the Destination Address field in the IPv4 packet header, except that the field contains a 128-bit destination address for IPv6 instead of a 32-bit destination address for IPv4. |
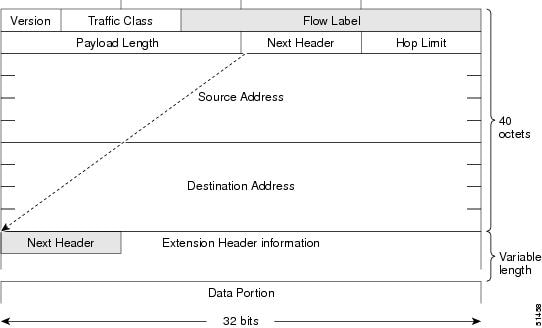
IPv6 Extension Headers
Optional extension headers and the data portion of the packet are after the eight fields of the base IPv6 packet header. If present, each extension header is aligned to 64 bits. There is no fixed number of extension headers in an IPv6 packet. Each extension header is identified by the Next Header field of the previous header. Typically, the final extension header has a Next Header field of a transport-layer protocol, such as TCP or UDP. The following figure shows the IPv6 extension header format.
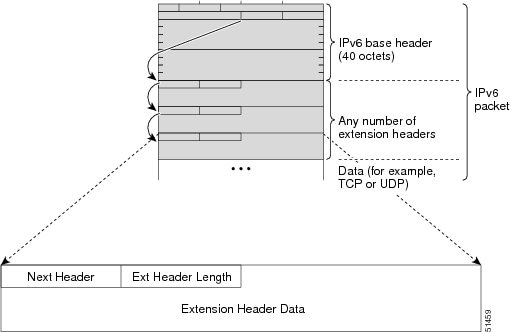
The table below lists the extension header types and their Next Header field values.
Header Type | Next Header Value | Description |
---|---|---|
Hop-by-hop options |
0 |
Header that is processed by all hops in the path of a packet. When present, the hop-by-hop options header always follows immediately after the base IPv6 packet header. |
Destination options |
60 |
Header that can follow any hop-by-hop options header. The header is processed at the final destination and at each visited address specified by a routing header. |
Routing |
43 |
Header that is used for source routing. |
Fragment |
44 |
Header that is used when a source fragments a packet that is larger than the maximum transmission unit (MTU) for the path between itself and a destination. The Fragment header is used in each fragmented packet. |
Authentication |
51 |
Header that is used to provide connectionless integrity and data origin authentication for packets. |
Encapsulation Security Payload |
50 |
All information following this header is encrypted. |
Mobility |
135 |
Header that is used in support of Mobile IPv6 service. |
Host Identity Protocol |
139 |
Header that is used for Host Identity Protocol version 2 (HIPv2), which provides secure methods for IP multihoming and mobile computing. |
Shim6 |
140 |
Header that is used for IP multihoming, which allows a host to be connected to multiple networks. |
Upper layer headers |
6 (TCP) 17 (UDP) |
Headers that are used inside a packet to transport the data. The two main transport protocols are TCP and UDP. |
![]() Note |
Some switch models support only a subset of IPv6 extension header types. The following list shows the extension header types that are supported by Cisco Nexus 3600 Platform Switches (N3K-C36180YC-R and N3K-C3636C-R) and by Cisco Nexus 9504 and 9508 modular chassis with these line cards: N9K-X9636C-R, N9K-X9636Q-R, N9K-X9636C-RX, and N9K-X96136YC-R. Supported: Destination options (60), Routing (43), Fragment (44), Mobility (135), Host Identity Protocol (HIP) (139), Shim6 (140). Not supported: Hop-by-hop options (0), Encapsulation Security Payload (50), Authentication Header (51), and experimental (253 and 254). Beginning with Cisco NX-OS Release 9.3(7), if you configure an IPv6 ACL on the devices listed here, you must include a new rule for the disposition of IPv6 packets that include extension headers. For the necessary configuration procedure, see "Configuring an ACL for IPv6 Extension Headers" in NX-OS Release 9.3(x) or later of the Cisco Nexus 9000 Series NX-OS Security Configuration Guide. |
DNS for IPv6
IPv6 supports DNS record types that are supported in the DNS name-to-address and address-to-name lookup processes. The DNS record types support IPv6 addresses (see the table).
![]() Note |
IPv6 also supports the reverse mapping of IPv6 addresses to DNS names. |
Record Type | Description | Format |
---|---|---|
AAAA |
Maps a hostname to an IPv6 address. (Equivalent to an A record in IPv4.) |
www.abc.test AAAA 3FFE:YYYY:C18:1::2 |
PTR |
Maps an IPv6 address to a hostname. (Equivalent to a PTR record in IPv4.) |
2.0.0.0.0.0.0.0.0.0.0.0.0.0.0.0.1.0.0.0.8.1.c.0.y.y.y.y.e.f.f.3.ip6.int PTR www.abc.test |
Path MTU Discovery for IPv6
As in IPv4, you can use path MTU discovery in IPv6 to allow a host to dynamically discover and adjust to differences in the MTU size of every link along a data path. In IPv6, however, fragmentation is handled by the source of a packet when the path MTU of one link along a given data path is not large enough to accommodate the size of the packets. Having IPv6 hosts handle packet fragmentation saves IPv6 router processing resources and helps IPv6 networks run more efficiently. Once the path MTU is reduced by the arrival of an ICMP Too Big message, Cisco NX-OS retains the lower value. The connection does not increase the segment size to gauge the throughput.
![]() Note |
In IPv6, the minimum link MTU is 1280 octets. We recommend that you use an MTU value of 1500 octets for IPv6 links. |
CDP IPv6 Address Support
You can use the Cisco Discovery Protocol (CDP) IPv6 address support for the neighbor information feature to transfer IPv6 addressing information between two Cisco devices. Cisco Discovery Protocol support for IPv6 addresses provides IPv6 information to network management products and troubleshooting tools.
ICMP for IPv6
You can use ICMP in IPv6 to provide information about the health of the network. ICMPv6, the version that works with IPv6, reports errors if packets cannot be processed correctly and sends informational messages about the status of the network. For example, if a router cannot forward a packet because it is too large to be sent out on another network, the router sends out an ICMPv6 message to the originating host. Additionally, ICMP packets in IPv6 are used in IPv6 neighbor discovery and path MTU discovery. The path MTU discovery process ensures that a packet is sent using the largest possible size that is supported on a specific route.
A value of 58 in the Next Header field of the base IPv6 packet header identifies an IPv6 ICMP packet. The ICMP packet follows all the extension headers and is the last piece of information in the IPv6 packet.Within the IPv6 ICMP packets, the ICMPv6 Type and ICMPv6 Code fields identify IPv6 ICMP packet specifics, such as the ICMP message type. The value in the Checksum field is computed by the sender and checked by the receiver from the fields in the IPv6 ICMP packet and the IPv6 pseudo header.
![]() Note |
The IPv6 header does not have a checksum. But a checksum on the transport layer can determine if packets have not been delivered correctly. All checksum calculations that include the IP address in the calculation must be modified for IPv6 to accommodate the new 128-bit address. A checksum is generated using a pseudo header. |
The ICMPv6 Payload field contains error or diagnostic information that relates to IP packet processing. The following figure shows the IPv6 ICMP packet header format.

IPv6 Neighbor Discovery
You can use the IPv6 Neighbor Discovery Protocol (NDP) to determine whether a neighboring router is reachable. IPv6 nodes use neighbor discovery to determine the addresses of nodes on the same network (local link), to find neighboring routers that can forward their packets, to verify whether neighboring routers are reachable or not, and to detect changes to link-layer addresses. NDP uses ICMP messages to detect whether packets are sent to neighboring routers that are unreachable.
IPv6 Neighbor Solicitation Message
A node sends a neighbor solicitation message, which has a value of 135 in the Type field of the ICMP packet header, on the local link when it wants to determine the link-layer address of another node on the same local link (see figure below). The source address is the IPv6 address of the node that sends the neighbor solicitation message. The destination address is the solicited-node multicast address that corresponds to the IPv6 address of the destination node. The neighbor solicitation message also includes the link-layer address of the source node.

After receiving the neighbor solicitation message, the destination node replies by sending a neighbor advertisement message, which has a value of 136 in the Type field of the ICMP packet header, on the local link. The source address is the IPv6 address of the node (the IPv6 address of the node interface that sends the neighbor advertisement message). The destination address is the IPv6 address of the node that sends the neighbor solicitation message. The data portion includes the link-layer address of the node that sends the neighbor advertisement message.
After the source node receives the neighbor advertisement, the source node and destination node can communicate.
Neighbor solicitation messages can verify the reachability of a neighbor after a node identifies the link-layer address of a neighbor. When a node wants to verify the reachability of a neighbor, it uses the destination address in a neighbor solicitation message as the unicast address of the neighbor.
Neighbor advertisement messages are also sent when there is a change in the link-layer address of a node on a local link. When there is a change, the destination address for the neighbor advertisement is the all-nodes multicast address.
Neighbor unreachability detection identifies the failure of a neighbor or the failure of the forward path to the neighbor and is used for all paths between hosts and neighboring nodes (hosts or routers). Neighbor unreachability detection is performed for neighbors to which only unicast packets are being sent and is not performed for neighbors to which multicast packets are being sent.
A neighbor is considered reachable when a positive acknowledgment is returned from the neighbor (indicating that packets previously sent to the neighbor have been received and processed). A positive acknowledgment-from an upper-layer protocol (such as TCP)-indicates that a connection is making forward progress (reaching its destination). If packets are reaching the peer, they are also reaching the next-hop neighbor of the source. Forward progress is also a confirmation that the next-hop neighbor is reachable.
For destinations that are not on the local link, forward progress implies that the first-hop router is reachable. When acknowledgments from an upper-layer protocol are not available, a node probes the neighbor using unicast neighbor solicitation messages to verify that the forward path is still working. The return of a solicited neighbor advertisement message from the neighbor is a positive acknowledgment that the forward path is still working (neighbor advertisement messages that have the solicited flag set to a value of 1 are sent only in response to a neighbor solicitation message). Unsolicited messages confirm only the one-way path from the source to the destination node; solicited neighbor advertisement messages indicate that a path is working in both directions.
![]() Note |
A neighbor advertisement message that has the solicited flag set to a value of 0 is not considered as a positive acknowledgment that the forward path is still working. |
Neighbor solicitation messages are also used in the stateless autoconfiguration process to verify the uniqueness of unicast IPv6 addresses before the addresses are assigned to an interface. Duplicate address detection is performed first on a new, link-local IPv6 address before the address is assigned to an interface (the new address remains in a tentative state while duplicate address detection is performed). A node sends a neighbor solicitation message with an unspecified source address and a tentative link-local address in the body of the message. If another node is already using that address, the node returns a neighbor advertisement message that contains the tentative link-local address. If another node is simultaneously verifying the uniqueness of the same address, that node also returns a neighbor solicitation message. If no neighbor advertisement messages are received in response to the neighbor solicitation message and no neighbor solicitation messages are received from other nodes that are attempting to verify the same tentative address, the node that sent the original neighbor solicitation message considers the tentative link-local address to be unique and assigns the address to the interface.
IPv6 Stateless Autoconfiguration
All interfaces on IPv6 nodes must have a link-local address, which is usually automatically configured from the identifier for an interface and the link-local prefix FE80::/10. A link-local address enables a node to communicate with other nodes on the link and can be used to further configure the node.
IPv6 Stateless Address Autoconfiguration (SLAAC) is performed only on a management interface. For example, when SLAAC is enabled on a management interface, it generates a Link Local Address (LLA) and performs a Duplicate Address Detection (DAD) on link local address. After the successful duplicate address detection process, the interface transmits ICMPv6 Router Solicitation (RS) packets. The upstream router that receives the RS packets responds back with an ICMPv6 Router Advertisement (RA). The RA packet will have a prefix TLV option that carries the subnet in which the downstream NX-OS Switch auto-generates the address, using the MAC information of the interface and the advertised prefix in RA packet. The Cisco NX-OS Switch auto-generates address in EUI-64 format and performs DAD on the new auto-generated addresses.
IPv6 addresses are assigned to an interface for a specific length of time. Each address has a lifetime that indicates how long the address is attached to an interface. The TLV prefix in the RA packet sent from the upstream router contain information about valid lifetime and preferred lifetime. The addresses that are assigned to an interface goes through two distinct phases. Initially, an address goes to a preferred state which means the address is not restricted for using in arbitrary communication. The address becomes deprecated state when the current interface binding becomes invalid. In a deprecated state, the use of the address is discouraged, not necessarily forbidden. Only applications that would have difficulty in switching to another address without a service disruption must use a deprecated address.
IPv6 Router Advertisement Message
Router advertisement (RA) messages, which have a value of 134 in the Type field of the ICMP packet header, are periodically sent out to each configured interface of an IPv6 router. For stateless autoconfiguration to work properly, the advertised prefix length in RA messages must always be 64 bits.
The RA messages are sent to the all-nodes multicast address (see the following figure).

The RA messages are sent to the all-nodes multicast address.
RA messages typically include the following information:
-
One or more onlink IPv6 prefixes that nodes on the local link can use to automatically configure their IPv6 addresses
-
Life-time information for each prefix included in the advertisement
-
Sets of flags that indicate the type of autoconfiguration (stateless or stateful) that can be completed
-
Default router information (whether the router sending the advertisement should be used as a default router and, if so, the amount of time in seconds that the router should be used as a default router)
-
Additional information for hosts, such as the hop limit and MTU that a host should use in packets that it originates
RAs are also sent in response to router solicitation messages. Router solicitation messages, which have a value of 133 in the Type field of the ICMP packet header, are sent by hosts at system startup so that the host can immediately autoconfigure without needing to wait for the next scheduled RA message. The source address is usually the unspecified IPv6 address (0:0:0:0:0:0:0:0). If the host has a configured unicast address, the unicast address of the interface that sends the router solicitation message is used as the source address in the message. The destination address is the all-routers multicast address with a scope of the link. When an RA is sent in response to a router solicitation, the destination address in the RA message is the unicast address of the source of the router solicitation message.
You can configure the following RA message parameters:
-
The time interval between periodic RA messages
-
The router life-time value, which indicates the usefulness of a router as the default router (for use by all nodes on a given link)
-
The network prefixes in use on a given link
-
The time interval between neighbor solicitation message retransmissions (on a given link)
-
The amount of time that a node considers a neighbor reachable (for use by all nodes on a given link)
The configured parameters are specific to an interface. The sending of RA messages (with default values) is automatically enabled on Ethernet interfaces. For other interface types, you must enter the no ipv6 nd suppress-ra command to send RA messages. You can disable the RA message feature on individual interfaces by entering the ipv6 nd suppress-ra command.
IPv6 Neighbor Redirect Message
Routers send neighbor redirect messages to inform hosts of better first-hop nodes on the path to a destination. A value of 137 in the Type field of the ICMP packet header identifies an IPv6 neighbor redirect message.

![]() Note |
A router must be able to determine the link-local address for each of its neighboring routers in order to ensure that the target address (the final destination) in a redirect message identifies the neighbor router by its link-local address. For static routing, you should specify the address of the next-hop router using the link-local address of the router. For dynamic routing, you must configure all IPv6 routing protocols to exchange the link-local addresses of neighboring routers. |
After forwarding a packet, a router sends a redirect message to the source of the packet under the following circumstances:
-
The destination address of the packet is not a multicast address.
-
The packet was not addressed to the router.
-
The packet is about to be sent out the interface on which it was received.
-
The router determines that a better first-hop node for the packet resides on the same link as the source of the packet.
-
The source address of the packet is a global IPv6 address of a neighbor on the same link or a link-local address.
IPv6 Anycast Addresses
An anycast address is an address that is assigned to a set of interfaces that belong to different nodes. A packet sent to an anycast address is delivered to the closest interface—as defined by the routing protocols in use—identified by the anycast address. Anycast addresses are syntactically indistinguishable from unicast addresses because anycast addresses are allocated from the unicast address space. Assigning a unicast address to more than one interface turns a unicast address into an anycast address. You must configure the nodes to which the anycast address belongs to recognize that the address is an anycast address.
![]() Note |
Anycast addresses can be used only by a router, not a host. Anycast addresses cannot be used as the source address of an IPv6 packet. |
The following figure shows the format of the subnet router anycast address; the address has a prefix concatenated by a series of zeros (the interface ID). The subnet router anycast address can be used to reach a router on the link that is identified by the prefix in the subnet router anycast address.

IPv6 Multicast Addresses
An IPv6 multicast address is an IPv6 address that has a prefix of FF00::/8 (1111 1111). An IPv6 multicast address is an identifier for a set of interfaces that belong to different nodes. A packet sent to a multicast address is delivered to all interfaces identified by the multicast address. The second octet following the prefix defines the lifetime and scope of the multicast address. A permanent multicast address has a lifetime parameter equal to 0; a temporary multicast address has a lifetime parameter equal to 1. A multicast address that has the scope of a node, link, site, or organization, or a global scope, has a scope parameter of 1, 2, 5, 8, or E, respectively. For example, a multicast address with the prefix FF02::/16 is a permanent multicast address with a link scope. The following figure shows the format of the IPv6 multicast address.
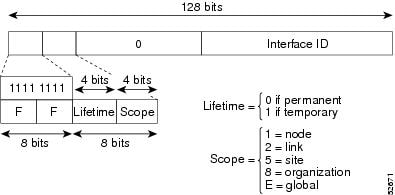
IPv6 nodes (hosts and routers) are required to join (where received packets are destined for) the following multicast groups:
-
All-nodes multicast group FF02:0:0:0:0:0:0:1 (the scope is link-local)
-
Solicited-node multicast group FF02:0:0:0:0:1:FF00:0000/104 for each of its assigned unicast and anycast addresses
IPv6 routers must also join the all-routers multicast group FF02:0:0:0:0:0:0:2 (the scope is link-local).
The solicited-node multicast address is a multicast group that corresponds to an IPv6 unicast or anycast address. IPv6 nodes must join the associated solicited-node multicast group for every unicast and anycast address to which they are assigned. The IPv6 solicited-node multicast address has the prefix FF02:0:0:0:0:1:FF00:0000/104 concatenated with the 24 low-order bits of a corresponding IPv6 unicast or anycast address (see the figure below). For example, the solicited-node multicast address that corresponds to the IPv6 address 2037::01:800:200E:8C6C is FF02::1:FF0E:8C6C. Solicited-node addresses are used in neighbor solicitation messages.
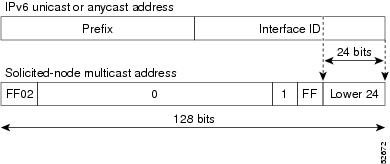
![]() Note |
IPv6 has no broadcast addresses. IPv6 multicast addresses are used instead of broadcast addresses. |
LPM Routing Modes
By default, Cisco NX-OS programs routes in a hierarchical fashion to allow for the longest prefix match (LPM) on the device. However, you can configure the device for different routing modes to support more LPM route entries.
The following tables list the LPM routing modes that are supported on Cisco Nexus 9000 Series switches.
LPM Routing Mode |
CLI Command |
---|---|
Default system routing mode |
|
LPM dual-host routing mode |
system routing template-dual-stack-host-scale |
LPM heavy routing mode |
system routing template-lpm-heavy |
![]() Note |
Cisco Nexus 9200 platform switches do not support the system routing template-lpm-heavy mode for IPv4 Multicast routes. Make sure to reset LPM's maximum limit to 0. |
LPM Routing Mode |
Broadcom T2 Mode |
CLI Command |
---|---|---|
Default system routing mode |
3 |
|
ALPM routing mode |
4 |
system routing max-mode l3 |
LPM Routing Mode |
CLI Command |
---|---|
LPM dual-host routing mode |
system routing template-dual-stack-host-scale |
LPM heavy routing mode |
system routing template-lpm-heavy |
LPM Internet-peering mode |
system routing template-internet-peering |
LPM Routing Mode |
Broadcom T2 Mode |
CLI Command |
||
---|---|---|---|---|
Default system routing mode |
3 (for line cards); 4 (for fabric modules) |
|||
Max-host routing mode |
2 (for line cards); 3 (for fabric modules) |
system routing max-mode host |
||
Nonhierarchical routing mode |
3 (for line cards); 4 with max-l3-mode option (for line cards) |
system routing non-hierarchical-routing [max-l3-mode] |
||
64-bit ALPM routing mode |
Submode of mode 4 (for fabric modules) |
system routing mode hierarchical 64b-alpm |
||
LPM heavy routing mode |
system routing template-lpm-heavy
|
|||
LPM Internet-peering mode |
system routing template-internet-peering
|
|||
LPM dual-host routing mode |
LPM Routing Mode |
CLI Command |
---|---|
LPM Internet-peering mode |
system routing template-internet-peering (Cisco NX-OS release 9.3(1) and later) |
Host to LPM Spillover
Beginning with Cisco NX-OS Release 7.0(3)I5(1), host routes can be stored in the LPM table in order to achieve a larger host scale. In ALPM mode, the switch allows fewer host routes. If you add more host routes than the supported scale, the routes that are spilled over from the host table take the space of the LPM routes in the LPM table. The total number of LPM routes allowed in that mode is reduced by the number of host routes stored. This feature is supported on Cisco Nexus 9300 and 9500 platform switches.
In the default system routing mode, Cisco Nexus 9300 platform switches are configured for higher host scale and fewer LPM routes, and the LPM space can be used to store more host routes. For Cisco Nexus 9500 platform switches, only the default system routing and nonhierarchical routing modes support this feature on line cards. Fabric modules do not support this feature.